Landfarming
Landfarming is a well proven ex-situ bioremediation technology that has been successfully used since the 1980s for treating petroleum impacted soils/sediments, drill cuttings, low brine drilling fluids, oily sludges, tank bottoms and pit sludges. The material to be treated is incorporated into surface soil. Naturally occurring microbes in the soil and waste material transform the organic contaminants to carbon dioxide, water and biomass ([1][2][3]). Maintaining optimum soil conditions for rapid biodegradation of organic contaminants can help meet cleanup goals within a reasonable timeframe.
Related Article(s):
CONTRIBUTOR(S): Dr. Roopa Kamath, Sara McMillen, Rene Bernier, and Deyuan Kong
Key Resource(s):
- Bioremediation using the land treatment concept.[1]
- Biotreating E&P Wastes: Lessons Learned from 1992-2003[2].
Introduction
During landfarming, the waste materials are typically placed as a layer on the ground surface with variable thickness. The waste is then tilled and amended with nutrients to enhance biodegradation by naturally occurring bacteria (Figure 1). Fertilizers such as urea and triple superphosphate (TSP) are used to provide nitrogen and phosphate necessary for biodegradation. Reduction in hydrocarbon concentrations can be expected within a span of weeks to months, depending on the initial concentration and composition of hydrocarbons, and whether the soil conditions are optimized for biodegradation. Once cleanup goals have been achieved, the treated material can be i) re-used in construction activity such as berms, landfill cover, backfill, regrading, or for agricultural purposes, ii) disposed at a landfill and/or iii) left in place and revegetated, depending on local regulations or site-specific considerations.
Landfarming is a low-cost technology. Facilities are simple to construct and easy to operate. Standard construction and farming equipment can be used to move soils to the land treatment facility, to amend the soils with fertilizer, to apply water to the soils and to till the soils (e.g. excavator, plow, rotovator, water truck). Figures 2 through 4 show examples of equipment used at a typical landfarming facility.
Some of the disadvantages of the technology are:
- It requires a large land area for treatment.
- There may be regulatory limitations on wastes that can be treated by landfarming. For example, U.S. regulations prevent landfarming soil impacted with hazardous wastes such as motor oil, hydraulic oil, and solvents.
- It may not be effective for highly impacted soils or soils impacted with severely degraded hydrocarbons (e.g. if soils contain >8% w/w petroleum hydrocarbons after spreading).
- Although landfarming is effective for reducing hydrocarbon concentrations, it is not effective for reducing concentrations of other oil field waste components, such as elevated concentrations of metals, salt or wastes containing naturally occurring radioactive materials (NORM).
- Concentration reductions >95% or final concentrations <0.1% may not be successfully obtained based on the extent impacted and nature of the hydrocarbons.
- Dust and vapor emissions may pose air quality concerns.
Suitability of Wastes for Landfarming
Landfarming has been successfully used to reduce hydrocarbon concentrations in soils impacted with kerosene, diesel, jet fuel, and crude oil. It has also been successfully used to treat oil-based drilling wastes (drill cutting, low brine drilling), oily sludge, tank bottoms and pit sludges.
Wastes may not be suitable for landfarming for a number of reasons:
- There may be regulatory or permit limitations. For instance, in the United States, wastes that are not exempted under RCRA [4] such as motor oil, hydraulic oil, and solvents cannot be treated by landfarming by law.
- Wastes unsuitable by their composition such as solid, non-spreadable paraffins from pig trap scrapings from the maintenance of pipelines, or highly weathered wastes, or asphaltic wastes
- Wastes containing contaminants that do not biodegrade: e.g., NORM, metals or salt.
Biodegradation Potential
The initial concentration and composition of hydrocarbons strongly influences the biodegradation potential of a waste.
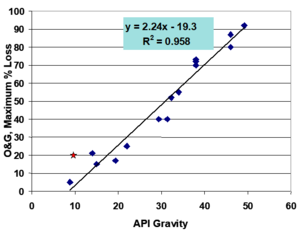
- Wastes with >5% (w/w) of hydrocarbons may have physical and chemical characteristics that hinder biodegradation. For example, oily wastes tend to repel water, and can be poorly aerated. This can hinder or inhibit biodegradation.
- The molecular structure and molecular weight of hydrocarbons in the waste may make them either more or less susceptible to attack by microorganisms. Typically, aliphatic hydrocarbons are more readily biodegraded than aromatic hydrocarbons. Structurally more complex molecules such as isoprenoids and steranes are relatively recalcitrant to biodegradation. For crude oils, the API gravity is a reliable indicator of the composition of a crude oil and by extension, a good predictor of the biodegradation potential of a crude oil (see Figure 5)[5]. For example, a crude oil with API gravity of 40 (by virtue of its composition) may degrade by 75% within 4 weeks. A crude oil with API gravity of 25 may only degrade by 55%, and a crude oil with API gravity of 10 may not degrade more than 10% within that same timeframe.
This same correlation can be used to determine the maximum initial hydrocarbon concentration that can be treated using conventional landfarming within 4 weeks and/or the maximum endpoint achievable for a given waste material.
Using analytical methods such as gas chromatography to determine the quantity and composition of organic contaminants in a waste may be useful in determining biodegradation potential. Lighter ends (lower boiling point components that elute earlier in the chromatogram) will typically degrade faster than heavier end components.
- The extent of weathering (volatilization and biodegradation) may influence the rate and extent of biodegradation. Fresh crude oils with API gravity >30 are generally readily biodegradable; however, after extensive weathering as might be encountered at an old spill site, the oil may not be very biodegradable as most of the more volatile and biodegradable fractions of hydrocarbon have already disappeared. It is recommended that feasibility evaluations include hydrocarbon analysis of wastes using gas chromatography (GC) to assess the extent of weathering. (Figures 6a and 6b)
- Light refined products, such as diesel and jet fuels, are highly biodegradable, similar to crude oils with API gravity >40. Heavy refined products like Bunker C or heavy fuel oils will biodegrade at rates similar to low API-gravity crude oils.
Facility Design and Operation
Landfarming facilities are designed to prevent impacts to groundwater and surface water. The facilities typically include an impermeable or low permeability liner for the treatment pad to prevent leaching of chemical constituents from the treatment waste to the groundwater, and berms/dikes to prevent storm water runoff. (See Figure 7.)
Successful landfarm operation requires maintaining optimum conditions in the soil/waste materials (moisture, oxygen, pH and nutrient levels) to enhance biodegradation of petroleum hydrocarbons (see Table 1). Before beginning landfarm operations, the waste and the soil at the landfarm site should be characterized to establish the soil baseline characteristics and the need for soil additives such as agricultural lime for pH correction.
Parameter | Optimum Condition |
---|---|
Initial HC Concentration | 1% - 5% by weight in dry soil |
pH | 6 – 8.5. Agricultural lime can be added to increase soil pH. |
Aeration/Tilling | Initially and every 2 – 4 weeks. |
Moisture Content | 60% – 80% of water holding capacity (field capacity). |
Salt Content | Electrical conductance of leachate <30 mS/cm (mhos/cm). Preferably <4 mS/cm for maximum reuse potential after cleanup. Ocean water has an electrical conductance of ~50 mS/cm. |
Nutrients |
|
Maintaining optimum conditions for biodegradation is the key to achieving target cleanup goals within a reasonable timeframe. Figure 8 is an illustration of the effect of tilling and adding fertilizer on the rate of degradation of petroleum hydrocarbons.
- Fertilizer: Fertilizers can be added either manually or with mechanical spreaders. During landfarming, the nutrient conditions should be monitored, and additional fertilizer should be added if the concentration falls below action levels. Care should be taken not to add excess nutrients as it can result in fertilizer runoff and/or inhibit biodegradation.
- Moisture: Moisture should be maintained in the landfarm between 60 and 80% of the water holding capacity (field capacity) of the soil (roughly 15%-24% moisture by weight for most soils). For soils with less than 20% water holding capacity, some form of irrigation will likely be required. For water saturated soils, no tilling should be performed while the water content is high since this can impair soil texture. Irrigation water should be fresh. Low quality water may result in concentration of salt and subsequent inhibition of biotreatment.
- Aeration: Locally available agricultural equipment is most often used for tilling soils to break up clumps of clay and uniformly mix in amendments such as fertilizers, soil conditioners and water.
- Monitoring Needs: Commonly monitored parameters include hydrocarbon concentration, moisture content, nutrients, and pH. Parameters are monitored on a biweekly or monthly basis as needed to confirm that the biodegradation process is progressing smoothly.
Cleanup levels that can be attained
Landfarming has been successful in meeting cleanup goals for a variety of hydrocarbon impacted wastes within a reasonable timeframe (see Figure 9). It is important to note that reductions in concentrations of total petroleum hydrocarbons (TPH) that are greater than 95% of the original concentrations are difficult to achieve. It is also difficult to achieve a final concentration of TPH that is <0.1% of the dry weight of the soil. Cleanup goals for soils impacted with refined products such as gasoline may be lower than cleanup goals for crude oil based on risk to receptors and mobility of those products in the environment.
References
- ^ 1.0 1.1 U.S. Environmental Protection Agency (USEPA), 1993. Bioremediation using the land treatment concept. US Environmental Protection Agency. Office of Research and Development, Washington, D.C. Report.pdf
- ^ 2.0 2.1 U.S. Environmental Protection Agency (USEPA), 2003. Aerobic Biodegradation of Oily Wastes: A Field Guidance Book for Federal On-scene Coordinators, Version 1.0, October 2003. Region 6 South Central Response and Prevention Branch. Report.pdf
- ^ U.S. Environmental Protection Agency (USEPA), 2017. How to Evaluate Alternative Cleanup Technologies for Underground Storage Tank Sites: A Guide for Corrective Action Plan Reviewers, Chapter V: Landfarming , Land and Emergency Management 5401R, EPA 510-B-17-003. Report.pdf
- ^ U.S. Environmental Protection Agency (USEPA), 2002. Exemption of Oil and Gas Exploration and Production Wastes from Federal Hazardous Waste Regulations. Office of Solid Waste, EPA530-K-01-004. Report.pdf
- ^ 5.0 5.1 McMillen, S.J., Smart, R., Bernier, R. and Hoffmann, R.E., 2004, January. Biotreating E&P wastes: lessons learned from 1992-2003. In SPE International Conference on Health, Safety, and Environment in Oil and Gas Exploration and Production. Society of Petroleum Engineers. doi: 10.2118/86794-ms
See Also
- Hoffmann, R., Bernier, R., Smith, S. and McMillen, S., 2010, January. A Four-Step Biotreatability Protocol for Crude Oil Impacted Soil. In SPE International Conference on Health, Safety and Environment in Oil and Gas Exploration and Production. Society of Petroleum Engineers.
- Huesemann, M.H., 1994. Guidelines for land‐treating petroleum hydrocarbon‐contaminated soils. Soil and Sediment Contamination, 3(3), pp.299-318.
- Lukić, B., Panico, A., Huguenot, D., Fabbricino, M., van Hullebusch, E.D. and Esposito, G., 2017. A review on the efficiency of landfarming integrated with composting as a soil remediation treatment. Environmental Technology Reviews, 6(1), pp.94-116.
- Maila, M.P. and Cloete, T.E., 2004. Bioremediation of petroleum hydrocarbons through landfarming: Are simplicity and cost-effectiveness the only advantages?. Reviews in Environmental science and bio/Technology, 3(4), pp.349-360.