Difference between revisions of "User:Jhurley/sandbox"
(→Field Applications) |
(→Passive Samplers) |
||
Line 53: | Line 53: | ||
|style="width:700px; text-align:center;" |<big>'''''C<sub><small>animal</small></sub> '''=''' f<sub><small>lipid</small></sub> '''x''' K<sub><small>lipid-water</small></sub> '''x''' C<sub><small>polymer</small></sub> '''/''' K<sub><small>polymer-water</small></sub>'''''</big> | |style="width:700px; text-align:center;" |<big>'''''C<sub><small>animal</small></sub> '''=''' f<sub><small>lipid</small></sub> '''x''' K<sub><small>lipid-water</small></sub> '''x''' C<sub><small>polymer</small></sub> '''/''' K<sub><small>polymer-water</small></sub>'''''</big> | ||
|} | |} | ||
− | [[File: Gschwend1w2fig2a.PNG | thumb | 300px | Figure 2a. Plot of the initial concentrations of a PRC (green lines) in a polyethylene (PE) sheet inserted in a sediment showing constant concentration across the PE and zero concentration outside the PE. At the same time, a target contaminant of interest (red lines) initially has a constant concentration in the sediment outside the PE and zero concentration inside the PE.]][[File: Gschwend1w2fig2b.PNG | thumb | 300px | Figure 2b. After the PE has been deployed for a time, the PRC is depleted from the PE (green lines), especially near the surfaces contacting the sediment, and its concentration is building up outside the PE and diffusing away into the sediment. Meanwhile, the target chemical leaves the sediment and begins to diffuse into the PE (red lines). The "jumps" in concentration at the PE-sediment boundary reflect the equilibrium paritioning coefficient, ''K<sub>PE-sed</sub> = C<sub>PE</sub>/C<sub>sediment</sub>''.]] | + | [[File: Gschwend1w2fig2a.PNG | thumb | 300px | Figure 2a. Plot of the initial concentrations of a PRC (green lines) in a polyethylene (PE) sheet inserted in a sediment showing constant concentration across the PE and zero concentration outside the PE. At the same time, a target contaminant of interest (red lines) initially has a constant concentration in the sediment outside the PE and zero concentration inside the PE.]][[File: Gschwend1w2fig2b.PNG | thumb | 300px | Figure 2b. After the PE has been deployed for a time, the PRC is depleted from the PE (green lines), especially near the surfaces contacting the sediment, and its concentration is building up outside the PE and diffusing away into the sediment. Meanwhile, the target chemical leaves the sediment and begins to diffuse into the PE (red lines). The "jumps" in concentration at the PE-sediment boundary reflect the equilibrium paritioning coefficient, ''K<sub>PE-sed</sub> = C<sub>PE</sub> / C<sub>sediment</sub>''.]] |
==Performance Reference Compounds (PRCs)== | ==Performance Reference Compounds (PRCs)== |
Revision as of 23:01, 22 May 2021
Passive Sampling of Sediments
"Passive sampling" refers to a group of methods used to quantify the availability of organic contaminants to move between different media and/or to react in environmental systems such as indoor air, lake waters, or contaminated sediment beds. To do this, the passive sampling material is deployed in the environmental system and allowed to absorb chemicals of interest via diffusive transfers from the surroundings. Upon recovery of the passive sampler, the accumulated contaminants are measured, and the concentrations in the sampler are interpreted to infer the chemical concentrations in specific surrounding media like porewater in a sediment bed. Such data are then useful inputs for site assessments such as those seeking to quantify fluxes from contaminated sediment beds to overlying waters or to evaluate the risk of significant uptake into benthic infauna and the larger food web.
Related Article(s):
- Contaminated Sediments - Introduction
- In Situ Treatment of Contaminated Sediments with Activated Carbon
- Passive Sampling of Munitions Constituents
Contributor(s): Dr. Philip M. Gschwend
Key Resource(s):
- Validating the Use of Performance Reference Compounds in Passive Samplers to Assess Porewater Concentrations in Sediment Beds[1]
- In situ passive sampling of sediments in the Lower Duwamish Waterway Superfund site: Replicability, comparison with ex situ measurements, and use of data[2]
- Laboratory, Field, and Analytical Procedures for Using Passive Sampling in the Evaluation of Contaminated Sediments: User’s Manual[3]
Introduction
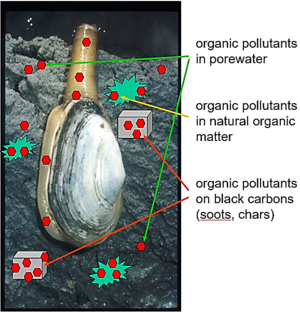
Environmental media such as sediments typically contain many different materials or phases, including liquid solutions (e.g. water, nonaqueous phase liquids like spilled oils) and diverse solids (e.g., quartz, aluminosilicate clays, and combustion-derived soots). Further, the chemical concentration in the porewater medium includes both molecules that are "truly dissolved" in the water and others that are associated with colloids in the porewater[4][5][6]. As a result, contaminant chemicals distribute among these diverse media (Figure 1) according to their affinity for each and the amount of each phase in the system[7][8][9][10][11]. As such, the chemical concentration in any one medium (e.g., truly dissolved in porewater) in a multi-material system like sediment is very hard to know from measures of the total sediment concentration, which unfortunately is the information typically found by analyzing for chemicals in sediment samples.
If an animal moves into this system, it will also accumulate the chemical in its tissues from the loads in all the other materials (Figure 1). This can lead to exposures of the chemical to other organisms, including humans, who may eat such animals. Predicting the quantity of contaminant in the animal requires knowledge of the relative affinities of the chemical for the animal versus the sediment materials. For example, if one knew the chemical's truly dissolved concentration in the porewater and could reasonably assume the chemical of interest in the animal has mostly accumulated in its lipids (as is often the case for very hydrophobic compounds), then one could estimate the chemical concentration in the animal (Canimal, typically in units of μg/kg animal wet weight) using a lipid-water partition coefficient, Klipid-water, typically in units of (μg/kg lipid)/(μg/L water), and the porewater concentration of the chemical (Cporewater, in μg/L) with Equation 1.
Equation 1. | Canimal = flipid x Klipid-water x Cporewater | |
where: | ||
flipid | is the fraction lipids contribute to the total wet weight of the animal (kg lipid/kg animal wet weight), and | |
Cporewater | is the freely dissolved contaminant concentration in the porewater surrounding the animal. |
While there is a great deal of information on the values of Klipid-water for many chemicals[12], it is often very inaccurate to estimate truly dissolved porewater concentrations from total sediment concentrations using assumptions about the affinity of those chemicals for the solids in the system[7]. Further, it is difficult to isolate porewater without colloids and/or measure the very low truly dissolved concentrations of hydrophobic contaminants of concern like polycyclic aromatic hydrocarbons (PAHs), polychlorinated biphenyls (PCBs), nonionic pesticides like dichlorodiphenyltrichloroethane (DDT), and polychlorinated dibenzo-p-dioxins (PCDDs)/ dibenzofurans (PCDFs)[13].
Passive Samplers
One approach to address this problem for contaminated sediments is to insert into the sediment billets of organic polymers like low density polyethylene (LDPE), polydimethylsiloxane (PDMS), or polyoxymethylene (POM) that can absorb such hydrophobic chemicals from their surroundings[14][15][16][17][18][19][2]. In this approach, the polymer is inserted in the sediment bed where it absorbs some of the contaminant load via the contaminant's diffusion into the polymer from the surroundings. When the polymer achieves sorptive equilibration with the sediments, the chemical concentration in the polymer, Cpolymer (μg/kg polymer), can be used to find the corresponding concentration in the porewater, Cporewater (μg/L), using a polymer-water partition coefficient, Kpolymer-water ((μg/kg polymer)/(μg/L water)), that has previously been found in laboratory testing[20][21], as shown in Equation 2.
Equation 2. | Cporewater = Cpolymer / Kpolymer-water |
Such “passive uptake” by the polymer also reflects the availability of the chemicals for transport to adjacent systems (e.g., overlying surface waters) and for uptake into organisms (e.g., bioaccumulation). Thus, one can use the porewater concentrations to estimate the biotic accumulation of the chemicals, too. For example, for the concentration in the animal equilibrated with the sediment, Canimal (μg/kg animal), would be found by combining Equations 1 and 2 to get Equation 3.
Equation 3. | Canimal = flipid x Klipid-water x Cpolymer / Kpolymer-water |
Performance Reference Compounds (PRCs)
Perhaps unsurprisingly, pollutants with low water solubility like PAHs, PCBs, etc. do not diffuse quickly through sediment beds. As a result, their accumulation in polymeric materials in sediments can take a long time to achieve equilibration[22][23][24]. This problem was recognized previously for passive samplers called semipermeable membrane devices (SPMDs, e.g. polyethylene bags filled with triolein[25]) that were deployed in surface waters. As a result, representative chemicals called performance reference compound (PRCs) were dosed inside the samplers before their deployment in the environment, and the PRCs' diffusive losses out of the SPMD could be used to quantify the fractional approach toward sampler-environmental surroundings equilibration[26][25]. A similar approach can be used for polymers inserted in sediment beds[22][1]. Commonly, isotopically labeled forms of the compounds of interest such as deuterated or 13C-labelled PAHs or PCBs are homogeneously impregnated into the polymers before their deployments. Upon insertion of the polymer into the sediment bed (or overlying waters or even air), the initially evenly distributed PRCs begin to diffuse out of the sampling polymer and into the surroundings (Figure 2).
Assuming the contaminants of interest undergo the same mass transfer restrictions limiting their rates of uptake into the polymer (e.g., diffusion through the sedimentary porous medium) that are also limiting transfers of the PRCs out of the polymer[22][1], then fractional losses of the PRCs during a particular deployment can be used to adjust the accumulated contaminant loads to what they would have been at equilibrium with their surroundings with Equation 4.
Equation 4. | C(∞)polymer = C(t)polymer / fPRC lost | |
where: | ||
fPRC lost | is the fraction of the PRC lost to outward diffusion, | |
C(∞)polymer | is the concentration of the contaminant in the polymer at equilibrium, and | |
C(t)polymer | is the concentration of the contaminant in the polymer after deployment time, t. |
Since investigators are commonly interested in many chemicals at the same time, it is impractical to have a PRC for each contaminant of interest. Instead, a representative set of PRCs is used to characterize the rates of polymer-environment exchange as a function of the PRCs' properties (e.g., diffusivities, partition coefficients), the sediments characteristics (e.g., porosity), and the nature of the polymer used (e.g., film thickness, affinity for the chemicals)[22][23]. The resulting mass transfer model fit can then be used to estimate the fractional approaches to equilibrium for many other contaminants, whose diffusive and partitioning properties are also known. And these fractions can be used to adjust the target chemical concentrations that have accumulated from the sediment into the same polymeric sampler to find the equilibrated results[1]. Finally, these equilibrated concentrations can be used in Eq. 2 to estimate truly dissolved contaminant concentrations in the sediment's porewater.
Field Applications
Polymeric materials can be deployed in sediment in various ways[3]. PDMS coatings can be incorporated into slotted silica rods called SPMEs (solid phase micro extraction devices), while thin sheets of polymers like LDPE or POM can be incorporated into sheet metal frames. In both cases, such hardware is used to insert the polymers into sediment beds (Figure 3).
Deployment of the assembled passive samplers can be accomplished via poles from a boat[1], by divers[2], or by attaching the samplers to a sampling platform lowered off a vessel[27]. Typically, the method used depends on the water depth. Small buoys on short lines, sometimes with associated water-sampling polymeric materials in mesh bags (see right panel of Figure 3), are attached to the samplers to facilitate the sampler recoveries. After recovery, the samplers are wiped to remove any adhering sediment, biofilm, or precipitates and returned to the laboratory for PRC and target contaminant analyses. The resulting measurements of the accumulated target chemical concentrations can be adjusted using the observed PRC losses and publicly available software programs[28][29].
Subsequently, since the passive sampling reveals the concentrations of contaminants in a sediment bed's porewater and the overlying bottom water[15], the data can be used to estimate bed-to-water column diffusive fluxes of contaminants[30][27] and bioirrigation-affected fluxes[31]. The data are also useful for assessing the tendency of the contaminants to accumulate in benthic organisms[32][33][34], and by extension into food webs that include such benthic species[35]. Furthermore, recent efforts have found that passive sampling observations can be used to infer in situ transformations of substances like nitro aromatic compounds[36] and DDT[37].
References
- ^ 1.0 1.1 1.2 1.3 1.4 Apell, J.N. and Gschwend, P.M., 2014. Validating the Use of Performance Reference Compounds in Passive Samplers to Assess Porewater Concentrations in Sediment Beds. Environmental Science and Technology, 48(17), pp. 10301-10307. DOI: 10.1021/es502694g
- ^ 2.0 2.1 2.2 Apell, J.N., and Gschwend, P.M., 2016. In situ passive sampling of sediments in the Lower Duwamish Waterway Superfund site: Replicability, comparison with ex situ measurements, and use of data. Environmental Pollution, 218, pp. 95-101. DOI: 10.1016/j.envpol.2016.08.023 Authors’ Manuscript
- ^ 3.0 3.1 Burgess, R.M., Kane Driscoll, S.B., Burton, A., Gschwend, P.M., Ghosh, U., Reible, D., Ahn, S., and Thompson, T., 2017. Laboratory, Field, and Analytical Procedures for Using Passive Sampling in the Evaluation of Contaminated Sediments: User’s Manual, EPA/600/R-16/357. SERDP/ESTCP and U.S. EPA, Office of Research and Development, Washington, DC 20460. Website Report.pdf
- ^ Brownawell, B.J., and Farrington, J.W., 1986. Biogeochemistry of PCBs in interstitial waters of a coastal marine sediment. Geochimica et Cosmochimica Acta, 50(1), pp. 157-169. DOI: 10.1016/0016-7037(86)90061-X Free download available from: US EPA.
- ^ Chin, Y.P., and Gschwend, P.M., 1992. Partitioning of Polycyclic Aromatic Hydrocarbons to Marine Porewater Organic Colloids. Environmental Science and Technology, 26(8), pp. 1621-1626. DOI: 10.1021/es00032a020
- ^ Achman, D.R., Brownawell, B.J., and Zhang, L., 1996. Exchange of Polychlorinated Biphenyls Between Sediment and Water in the Hudson River Estuary. Estuaries, 19(4), pp. 950-965. DOI: 10.2307/1352310 Free download available from: Academia.edu
- ^ 7.0 7.1 Gustafsson, Ö., Haghseta, F., Chan, C., MacFarlane, J., and Gschwend, P.M., 1996. Quantification of the Dilute Sedimentary Soot Phase: Implications for PAH Speciation and Bioavailability. Environmental Science and Technology, 31(1), pp. 203-209. DOI: 10.1021/es960317s
- ^ Luthy, R.G., Aiken, G.R., Brusseau, M.L., Cunningham, S.D., Gschwend, P.M., Pignatello, J.J., Reinhard, M., Traina, S.J., Weber, W.J., and Westall, J.C., 1997. Sequestration of Hydrophobic Organic Contaminants by Geosorbents. Environmental Science and Technology, 31(12), pp. 3341-3347. DOI: 10.1021/es970512m
- ^ Lohmann, R., MacFarlane, J.K., and Gschwend, P.M., 2005. Importance of Black Carbon to Sorption of Native PAHs, PCBs, and PCDDs in Boston and New York Harbor Sediments. Environmental Science and Technology, 39(1), pp.141-148. DOI: 10.1021/es049424+
- ^ Cornelissen, G., Gustafsson, Ö., Bucheli, T.D., Jonker, M.T., Koelmans, A.A., and van Noort, P.C., 2005. Extensive Sorption of Organic Compounds to Black Carbon, Coal, and Kerogen in Sediments and Soils: Mechanisms and Consequences for Distribution, Bioaccumulation, and Biodegradation. Environmental Science and Technology, 39(18), pp. 6881-6895. DOI: 10.1021/es050191b
- ^ Koelmans, A.A., Kaag, K., Sneekes, A., and Peeters, E.T.H.M., 2009. Triple Domain in Situ Sorption Modeling of Organochlorine Pesticides, Polychlorobiphenyls, Polyaromatic Hydrocarbons, Polychlorinated Dibenzo-p-Dioxins, and Polychlorinated Dibenzofurans in Aquatic Sediments. Environmental Science and Technology, 43(23), pp. 8847-8853. DOI: 10.1021/es9021188
- ^ Schwarzenbach, R.P., Gschwend, P.M., and Imboden, D.M., 2017. Environmental Organic Chemistry, 3rd edition. Ch. 16: Equilibrium Partitioning from Water and Air to Biota, pp. 469-521. John Wiley and Sons. ISBN: 978-1-118-76723-8
- ^ Hawthorne, S.B., Grabanski, C.B., Miller, D.J., and Kreitinger, J.P., 2005. Solid-Phase Microextraction Measurement of Parent and Alkyl Polycyclic Aromatic Hydrocarbons in Milliliter Sediment Pore Water Samples and Determination of KDOC Values. Environmental Science and Technology, 39(8), pp. 2795-2803. DOI: 10.1021/es0405171
- ^ Mayer, P., Vaes, W.H., Wijnker, F., Legierse, K.C., Kraaij, R., Tolls, J., and Hermens, J.L., 2000. Sensing Dissolved Sediment Porewater Concentrations of Persistent and Bioaccumulative Pollutants Using Disposable Solid-Phase Microextraction Fibers. Environmental Science and Technology, 34(24), pp. 5177-5183. DOI: 10.1021/es001179g
- ^ 15.0 15.1 Booij, K., Hoedemaker, J.R., and Bakker, J.F., 2003. Dissolved PCBs, PAHs, and HCB in Pore Waters and Overlying Waters of Contaminated Harbor Sediments. Environmental Science and Technology, 37(18), pp. 4213-4220. DOI: 10.1021/es034147c
- ^ Cornelissen, G., Pettersen, A., Broman, D., Mayer, P., and Breedveld, G.D., 2008. Field testing of equilibrium passive samplers to determine freely dissolved native polycyclic aromatic hydrocarbon concentrations. Environmental Toxicology and Chemistry, 27(3), pp. 499-508. DOI: 10.1897/07-253.1
- ^ Tomaszewski, J.E., and Luthy, R.G., 2008. Field Deployment of Polyethylene Devices to Measure PCB Concentrations in Pore Water of Contaminated Sediment. Environmental Science and Technology, 42(16), pp. 6086-6091. DOI: 10.1021/es800582a
- ^ Fernandez, L.A., MacFarlane, J.K., Tcaciuc, A.P., and Gschwend, P.M., 2009. Measurement of Freely Dissolved PAH Concentrations in Sediment Beds Using Passive Sampling with Low-Density Polyethylene Strips. Environmental Science and Technology, 43(5), pp. 1430-1436. DOI: 10.1021/es802288w
- ^ Arp, H.P.H., Hale, S.E., Elmquist Kruså, M., Cornelissen, G., Grabanski, C.B., Miller, D.J., and Hawthorne, S.B., 2015. Review of polyoxymethylene passive sampling methods for quantifying freely dissolved porewater concentrations of hydrophobic organic contaminants. Environmental Toxicology and Chemistry, 34(4), pp. 710-720. DOI: 10.1002/etc.2864 Free access article. Report.pdf
- ^ Lohmann, R., 2012. Critical Review of Low-Density Polyethylene’s Partitioning and Diffusion Coefficients for Trace Organic Contaminants and Implications for Its Use as a Passive Sampler. Environmental Science and Technology, 46(2), pp. 606-618. DOI: 10.1021/es202702y
- ^ Ghosh, U., Kane Driscoll, S., Burgess, R.M., Jonker, M.T., Reible, D., Gobas, F., Choi, Y., Apitz, S.E., Maruya, K.A., Gala, W.R., Mortimer, M., and Beegan, C., 2014. Passive Sampling Methods for Contaminated Sediments: Practical Guidance for Selection, Calibration, and Implementation. Integrated Environmental Assessment and Management, 10(2), pp. 210-223. DOI: 10.1002/ieam.1507 Free access article. Report.pdf
- ^ 22.0 22.1 22.2 22.3 Fernandez, L. A., Harvey, C.F., and Gschwend, P.M., 2009. Using Performance Reference Compounds in Polyethylene Passive Samplers to Deduce Sediment Porewater Concentrations for Numerous Target Chemicals. Environmental Science and Technology, 43(23), pp. 8888-8894. DOI: 10.1021/es901877a
- ^ 23.0 23.1 Lampert, D.J., Thomas, C., and Reible, D.D., 2015. Internal and external transport significance for predicting contaminant uptake rates in passive samplers. Chemosphere, 119, pp. 910-916. DOI: 10.1016/j.chemosphere.2014.08.063 Free download available from: Academia.edu
- ^ Apell, J.N., Tcaciuc, A.P., and Gschwend, P.M., 2016. Understanding the rates of nonpolar organic chemical accumulation into passive samplers deployed in the environment: Guidance for passive sampler deployments. Integrated Environmental Assessment and Management, 12(3), pp. 486-492. DOI: 10.1002/ieam.1697
- ^ 25.0 25.1 Huckins, J.N., Petty, J.D., Lebo, J.A., Almeida, F.V., Booij, K., Alvarez, D.A., Cranor, W.L., Clark, R.C., and Mogensen, B.B., 2002. Development of the Permeability/Performance Reference Compound Approach for In Situ Calibration of Semipermeable Membrane Devices. Environmental Science and Technology, 36(1), pp. 85-91. DOI: 10.1021/es010991w
- ^ Booij, K., Smedes, F., and van Weerlee, E.M., 2002. Spiking of performance reference compounds in low density polyethylene and silicone passive water samplers. Chemosphere 46(8), pp.1157-1161. DOI: 10.1016/S0045-6535(01)00200-4
- ^ 27.0 27.1 Fernandez, L.A., Lao, W., Maruya, K.A., White, C., Burgess, R.M., 2012. Passive Sampling to Measure Baseline Dissolved Persistent Organic Pollutant Concentrations in the Water Column of the Palos Verdes Shelf Superfund Site. Environmental Science and Technology, 46(21), pp. 11937-11947. DOI: 10.1021/es302139y
- ^ Gschwend, P.M., Tcaciuc, A.P., and Apell, J.N., 2014. Guidance Document: Passive PE Sampling in Support of In Situ Remediation of Contaminated Sediments – Passive Sampler PRC Calculation Software User’s Guide, US Department of Defense, Environmental Security Technology Certification Program Project ER-200915. Available from: ESTCP.
- ^ Thompson, J.M., Hsieh, C.H. and Luthy, R.G., 2015. Modeling Uptake of Hydrophobic Organic Contaminants into Polyethylene Passive Samplers. Environmental Science and Technology, 49(4), pp. 2270-2277. DOI: 10.1021/es504442s
- ^ Koelmans, A.A., Poot, A., De Lange, H.J., Velzeboer, I., Harmsen, J., and van Noort, P.C.M., 2010. Estimation of In Situ Sediment-to-Water Fluxes of Polycyclic Aromatic Hydrocarbons, Polychlorobiphenyls and Polybrominated Diphenylethers. Environmental Science and Technology, 44(8), pp. 3014-3020. DOI: 10.1021/es903938z
- ^ Apell, J.N., Shull, D.H., Hoyt, A.M., and Gschwend, P.M., 2018. Investigating the Effect of Bioirrigation on In Situ Porewater Concentrations and Fluxes of Polychlorinated Biphenyls Using Passive Samplers. Environmental Science and Technology, 52(8), pp. 4565-4573. DOI: 10.1021/acs.est.7b05809
- ^ Vinturella, A.E., Burgess, R.M., Coull, B.A., Thompson, K.M., and Shine, J.P., 2004. Use of Passive Samplers to Mimic Uptake of Polycyclic Aromatic Hydrocarbons by Benthic Polychaetes. Environmental Science and Technology, 38(4), pp. 1154-1160. DOI: 10.1021/es034706f
- ^ Yates, K., Pollard, P., Davies, I.M., Webster, L., and Moffat, C.F., 2011. Application of silicone rubber passive samplers to investigate the bioaccumulation of PAHs by Nereis virens from marine sediments. Environmental Pollution, 159(12), pp. 3351-3356. DOI: 10.1016/j.envpol.2011.08.038
- ^ Fernandez, L.A. and Gschwend, P.M., 2015. Predicting bioaccumulation of polycyclic aromatic hydrocarbons in soft-shelled clams (Mya arenaria) using field deployments of polyethylene passive samplers. Environmental Toxicology and Chemistry, 34(5), pp. 993-1000. DOI: 10.1002/etc.2892
- ^ von Stackelberg, K., Williams, M.A., Clough, J., and Johnson, M.S., 2017. Spatially explicit bioaccumulation modeling in aquatic environments: Results from 2 demonstration sites. Integrated Environmental Assessment and Management, 13(6), pp. 1023-1037. DOI: 10.1002/ieam.1927
- ^ Belles, A., Alary, C., Criquet, J., and Billon, G., 2016. A new application of passive samplers as indicators of in-situ biodegradation processes. Chemosphere, 164, pp. 347-354. DOI: 10.1016/j.chemosphere.2016.08.111
- ^ Tcaciuc, A.P., Borrelli, R., Zaninetta, L.M., and Gschwend, P.M., 2018. Passive sampling of DDT, DDE and DDD in sediments: accounting for degradation processes with reaction–diffusion modeling. Environmental Science: Processes and Impacts, 20(1), pp. 220-231. DOI: 10.1039/C7EM00501F Open access article available from: Royal Society of Chemistry.