Difference between revisions of "User:Debra Tabron/sandbox"
Debra Tabron (talk | contribs) |
Debra Tabron (talk | contribs) |
||
Line 5: | Line 5: | ||
'''Related Article(s):''' | '''Related Article(s):''' | ||
− | *[[Bioremediation – Anaerobic | In Situ Anaerobic Bioremediation]] | + | *[[Bioremediation – Anaerobic| In Situ Anaerobic Bioremediation]] |
*[[Biodegradation - Reductive Processes]] | *[[Biodegradation - Reductive Processes]] | ||
*[[Design Tool for Estimating Base Required during ERD]] | *[[Design Tool for Estimating Base Required during ERD]] | ||
Line 30: | Line 30: | ||
Table 1 shows the range and optimum pH for growth of bacteria that reduce PCE to TCE and cDCE. Zhuang and Pavlostathis (1995)<ref>Zhuang, P. and Pavlostathis, S.G., 1995. Effect of temperature, pH and electron donor on the microbial reductive dechlorination of chloroalkenes. Chemosphere, 31(6), pp.3537-3548. [https://doi.org/10.1016/0045-6535(95)00204-L doi:10.1016/0045-6535(95)00204-L]</ref> found that neutral pH was optimum for reductive dechlorination by a methanogenic mixed culture capable of dechlorinating PCE to VC. Vainberg et al. (2006)<ref name= "Vainberg2006"/> reported an optimum pH of 6.0-6.8 for dechlorination of PCE by the SDC-9<sup>™</sup> bioaugmentation culture. Dechlorination of PCE and TCE to cDCE can occur at pH down to 5.5<ref>Vainberg, S., Condee, C.W. and Steffan, R.J., 2009. Large-scale production of bacterial consortia for remediation of chlorinated solvent-contaminated groundwater. Journal of Industrial Microbiology & Biotechnology, 36(9), pp.1189-1197. [https://doi.org/10.1007/s10295-009-0600-5 doi: 10.1007/s10295-009-0600-5]</ref>. | Table 1 shows the range and optimum pH for growth of bacteria that reduce PCE to TCE and cDCE. Zhuang and Pavlostathis (1995)<ref>Zhuang, P. and Pavlostathis, S.G., 1995. Effect of temperature, pH and electron donor on the microbial reductive dechlorination of chloroalkenes. Chemosphere, 31(6), pp.3537-3548. [https://doi.org/10.1016/0045-6535(95)00204-L doi:10.1016/0045-6535(95)00204-L]</ref> found that neutral pH was optimum for reductive dechlorination by a methanogenic mixed culture capable of dechlorinating PCE to VC. Vainberg et al. (2006)<ref name= "Vainberg2006"/> reported an optimum pH of 6.0-6.8 for dechlorination of PCE by the SDC-9<sup>™</sup> bioaugmentation culture. Dechlorination of PCE and TCE to cDCE can occur at pH down to 5.5<ref>Vainberg, S., Condee, C.W. and Steffan, R.J., 2009. Large-scale production of bacterial consortia for remediation of chlorinated solvent-contaminated groundwater. Journal of Industrial Microbiology & Biotechnology, 36(9), pp.1189-1197. [https://doi.org/10.1007/s10295-009-0600-5 doi: 10.1007/s10295-009-0600-5]</ref>. | ||
− | |||
'''Table 1. Range and optimum pH for growth of pure cultures reducing PCE ''' | '''Table 1. Range and optimum pH for growth of pure cultures reducing PCE ''' | ||
− | {|- class="wikitable" style="float:left; margin: | + | {|- class="wikitable" style="float:left; margin: left; width: 65%;" |
|- | |- | ||
!style="background-color:#CEE0F2;" | Organism!!style="background-color:#CEE0F2;"|pH Range!!style="background-color:#CEE0F2;"| pH Optimum!!style="background-color:#CEE0F2;"|Reference | !style="background-color:#CEE0F2;" | Organism!!style="background-color:#CEE0F2;"|pH Range!!style="background-color:#CEE0F2;"| pH Optimum!!style="background-color:#CEE0F2;"|Reference | ||
Line 39: | Line 38: | ||
| ''Dehalobacter restrictus'' PER- K23 || 6.5 to 8.0 ||6.8 to 7.6 || Holliger et al., 1998<ref>Holliger, C., Hahn, D., Harmsen, H., Ludwig, W., Schumacher, W., Tindall, B., Vazquez, F., Weiss, N. and Zehnder, A.J., 1998. Dehalobacter restrictus gen. nov. and sp. nov., a strictly anaerobic bacterium that reductively dechlorinates tetra-and trichloroethene in an anaerobic respiration. Archives of Microbiology, 169(4), pp.313-321. [https://doi.org/10.1007/s002030050577 doi: 10.1007/s002030050577]</ref> | | ''Dehalobacter restrictus'' PER- K23 || 6.5 to 8.0 ||6.8 to 7.6 || Holliger et al., 1998<ref>Holliger, C., Hahn, D., Harmsen, H., Ludwig, W., Schumacher, W., Tindall, B., Vazquez, F., Weiss, N. and Zehnder, A.J., 1998. Dehalobacter restrictus gen. nov. and sp. nov., a strictly anaerobic bacterium that reductively dechlorinates tetra-and trichloroethene in an anaerobic respiration. Archives of Microbiology, 169(4), pp.313-321. [https://doi.org/10.1007/s002030050577 doi: 10.1007/s002030050577]</ref> | ||
|- | |- | ||
− | | ''Sulfurospirillum multivorans'' || 5.5 to 8.0 || 7.0 to 7.5|| Neumann et al., 1994<ref>Neumann, A., Scholz-Muramatsu, H. and Diekert, G., 1994. Tetrachloroethene metabolism of Dehalospirillum multivorans. Archives of Microbiology, 162(4), pp.295-301. ISSN: 0302-8933, 1432-072X [https://doi.org/10.1007/bf00301854 doi: 10.1007/BF00301854]</ref>; Scholz | + | | ''Sulfurospirillum multivorans'' || 5.5 to 8.0 || 7.0 to 7.5|| Neumann et al., 1994<ref>Neumann, A., Scholz-Muramatsu, H. and Diekert, G., 1994. Tetrachloroethene metabolism of Dehalospirillum multivorans. Archives of Microbiology, 162(4), pp.295-301. ISSN: 0302-8933, 1432-072X [https://doi.org/10.1007/bf00301854 doi: 10.1007/BF00301854]</ref>; Scholz-Muramatsu et al., 1995<ref>Scholz-Muramatsu, H., Neumann, A., Meßmer, M., Moore, E. and Diekert, G., 1995. Isolation and characterization of Dehalospirillum multivorans gen. nov., sp. nov., a tetrachloroethene-utilizing, strictly anaerobic bacterium. Archives of Microbiology, 163(1), pp.48-56. [https://doi.org/10.1007/bf00262203 doi: 10.1007/BF00262203]</ref>; Yang, 2017a<ref name= "Yang2017a"/> |
|- | |- | ||
| ''Desulfitobacterium dehalogenans'' JW/IU-DC-1 || 6.0 to 9.0 || 7.5 ||Utkin et al., 1994<ref>Utkin, I., Woese, C. and Wiegel, J., 1994. Isolation and characterization of Desulfitobacterium dehalogenans gen. nov., sp. nov., an anaerobic bacterium which reductively dechlorinates chlorophenolic compounds. International Journal of Systematic and Evolutionary Microbiology, 44(4), pp.612-619. [https://doi.org/10.1099/00207713-44-4-612 doi: 10.1099/00207713-44-4-612]</ref> | | ''Desulfitobacterium dehalogenans'' JW/IU-DC-1 || 6.0 to 9.0 || 7.5 ||Utkin et al., 1994<ref>Utkin, I., Woese, C. and Wiegel, J., 1994. Isolation and characterization of Desulfitobacterium dehalogenans gen. nov., sp. nov., an anaerobic bacterium which reductively dechlorinates chlorophenolic compounds. International Journal of Systematic and Evolutionary Microbiology, 44(4), pp.612-619. [https://doi.org/10.1099/00207713-44-4-612 doi: 10.1099/00207713-44-4-612]</ref> | ||
Line 58: | Line 57: | ||
|} | |} | ||
− | <BR CLEAR=" | + | <BR CLEAR="right"> |
==Impact on Dechlorination Rates== | ==Impact on Dechlorination Rates== | ||
Lacroix et al. (2014)<ref name= "Lacroix2014">Lacroix, E., Brovelli, A., Barry, D.A. and Holliger, C., 2014. Use of silicate minerals for pH control during reductive dechlorination of chloroethenes in batch cultures of different microbial consortia. Applied and environmental microbiology, pp.AEM-00493. [https://doi.org/10.1128/aem.00493-14 doi: 10.1128/AEM.00493-14]</ref> examined the effect of pH on dechlorination rates for several organohalide-respiring consortia including SL2-PCEa, SL2-PCEb, AQ-1, and PM. Dechlorination rates as a function of pH (R<sub>pH</sub>) were fit to the relationship. | Lacroix et al. (2014)<ref name= "Lacroix2014">Lacroix, E., Brovelli, A., Barry, D.A. and Holliger, C., 2014. Use of silicate minerals for pH control during reductive dechlorination of chloroethenes in batch cultures of different microbial consortia. Applied and environmental microbiology, pp.AEM-00493. [https://doi.org/10.1128/aem.00493-14 doi: 10.1128/AEM.00493-14]</ref> examined the effect of pH on dechlorination rates for several organohalide-respiring consortia including SL2-PCEa, SL2-PCEb, AQ-1, and PM. Dechlorination rates as a function of pH (R<sub>pH</sub>) were fit to the relationship. | ||
Line 65: | Line 64: | ||
where R<sub>max</sub> is the maximum dechlorination rate at the optimal pH (pH<sub>opt</sub>), and n and σ are empirical fitting parameters (Table 2). Graphs of relative dechlorination rate (R<sub>pH</sub> / R<sub>max</sub>) are shown in Figure 1. Dechlorination of PCE to cDCE can continue at pH below 5.5. However, dechlorination of cDCE to VC and VC to ethene is severely inhibited below a pH of 5.5. | where R<sub>max</sub> is the maximum dechlorination rate at the optimal pH (pH<sub>opt</sub>), and n and σ are empirical fitting parameters (Table 2). Graphs of relative dechlorination rate (R<sub>pH</sub> / R<sub>max</sub>) are shown in Figure 1. Dechlorination of PCE to cDCE can continue at pH below 5.5. However, dechlorination of cDCE to VC and VC to ethene is severely inhibited below a pH of 5.5. | ||
− | [[File:Borden1w2 Table 2.PNG| | + | [[File:Borden1w2 Table 2.PNG|left| thumbnail| 500 px| (from Lacroix et al. 2014)<ref name= "Lacroix2014"/> ]] |
+ | [[File:Borden1w2 Figure1.png|left|thumbnail| 600 px|Figure 1. Relative dechlorination rates (R<sub>pH</sub> /R<sub>max</sub>) versus pH for consortia SL2-PCEa, SL2-PCEb, AQ-1, and PM (adapted from Lacroix et al. 2014)<ref name= "Lacroix2014"/>. ]] | ||
− | + | <BR CLEAR="right"> | |
− | |||
==Low pH Tolerant Enrichments== | ==Low pH Tolerant Enrichments== | ||
Multiple researchers have attempted to develop enrichment cultures capable of complete dechlorination of PCE and TCE to ethene at low pH. However, to date, these attempts have had only modest success. | Multiple researchers have attempted to develop enrichment cultures capable of complete dechlorination of PCE and TCE to ethene at low pH. However, to date, these attempts have had only modest success. | ||
Line 88: | Line 87: | ||
==References== | ==References== | ||
<references /> | <references /> | ||
+ | |||
+ | ==See Also== | ||
+ | *[http://www.siremlab.com/kb-1-kb-1-plus/ Low pH Bioaugmentation Cultures] | ||
+ | *[https://www.serdp-estcp.org/Program-Areas/Environmental-Restoration/Contaminated-Groundwater/Emerging-Issues/ER-2129 Secondary Impacts of In Situ Remediation on Groundwater Quality and Post-Treatment Management Strategies] |
Revision as of 18:33, 24 July 2018
Organohalide-respiring bacteria are generally sensitive to pH. Reductive dechlorination of tetrachloroethene (PCE) to trichloroethene (TCE) and then to cis-1,2-dichloroethene(cDCE) can occur at a pH as low as 5.5. However, rates of cDCE reduction to vinyl chloride (VC) and then to ethene are reduced below a pH of 6.0. For efficient dechlorination to non-toxic end products, aquifer pH should be maintained above 6.0 during enhanced reductive dechlorination.
Related Article(s):
- In Situ Anaerobic Bioremediation
- Biodegradation - Reductive Processes
- Design Tool for Estimating Base Required during ERD
- pH Buffering in Aquifers
- Chlorinated Solvents
CONTRIBUTOR(S): Dr. Robert Borden, P.E.
Key Resource(s):
- Adaptation of a Dechlorinating Culture, KB-1, to Acidic Environments.[1]
- Organohalide respiration with chlorinated ethenes under low pH conditions][2].
- Resilience and recovery of Dehalococcoides mccartyi following low pH exposure[3]
Introduction
Enhanced reductive dechlorination (ERD) is commonly used to treat chlorinated solvents and related contaminants in groundwater by providing a fermentable organic substrate to serve as both an electron donor and a carbon source to stimulate microbially mediated reductive dechlorination[4][5][6]. During ERD, the pH may decline as hydrochloric acid (HCl) is produced during reductive dechlorination[7] [8] and carbonic acid and organic acids are produced by substrate fermentation. However, dechlorinating bacteria appear to be particularly sensitive to pH changes with dechlorination of cDCE and VC to ethene completely inhibited at a pH of 5.5[9][10][11][2], resulting in a significant decline in degradation rates[12][13].
Effect of pH on Bioremediation Processes
Many biological processes are sensitive to pH. Most microorganisms important for subsurface bioremediation function most efficiently in near neutral conditions[14]. Low pH can interfere with pH homeostasis or increase the solubility of toxic metals[15]. Microorganisms can expend cellular energy to maintain homeostasis, or conditions in the cytoplasm and periplasm may change in response to external changes in pH[16]. Some anaerobes have adapted to low pH conditions through alterations in carbon and electron flow, cellular morphology, membrane structure, and protein synthesis[14].
There is some evidence that organohalide-respiring bacteria are particularly sensitive to changes in pH. Pure cultures and consortia of organohalide-respiring microorganisms show highest dechlorination rates at circumneutral pH[2]. Reduction of cDCE to VC to ethene is primarily carried out by strains of Dehalococcoides mccartyi (Dhc)[17]. Growth and dechlorination by Dhc occurs between pH 6 and 8, with highest activity measured between pH 6.9 and 7.5[17]
Table 1 shows the range and optimum pH for growth of bacteria that reduce PCE to TCE and cDCE. Zhuang and Pavlostathis (1995)[18] found that neutral pH was optimum for reductive dechlorination by a methanogenic mixed culture capable of dechlorinating PCE to VC. Vainberg et al. (2006)[10] reported an optimum pH of 6.0-6.8 for dechlorination of PCE by the SDC-9™ bioaugmentation culture. Dechlorination of PCE and TCE to cDCE can occur at pH down to 5.5[19].
Table 1. Range and optimum pH for growth of pure cultures reducing PCE
Organism | pH Range | pH Optimum | Reference | ||||||||
---|---|---|---|---|---|---|---|---|---|---|---|
Dehalobacter restrictus PER- K23 | 6.5 to 8.0 | 6.8 to 7.6 | Holliger et al., 1998[20] | ||||||||
Sulfurospirillum multivorans | 5.5 to 8.0 | 7.0 to 7.5 | Neumann et al., 1994[21]; Scholz-Muramatsu et al., 1995[22]; Yang, 2017a[2] | ||||||||
Desulfitobacterium dehalogenans JW/IU-DC-1 | 6.0 to 9.0 | 7.5 | Utkin et al., 1994[23] | ||||||||
Desulfitobacterium sp. PCE-1 | 7.2 to 7.8 | 7.2 | Gerritse et al., 1996[24] | ||||||||
Desulfitobacterium dichloroeliminans DCA1 | 7.2 to 7.8 | Fogel et al., 2009[25] | |||||||||
Desulfuromonus chloroethenica TT4B | 6.5 to 7.4 | 7.4 | Krumholz el al., 1996[26];Krumholz, 1997[27] | ||||||||
Desulfomonile tiedjei DCB-1 | 6.5 to 7.8 | 6.8 to 7.0 | DeWeerd et al., 1990[28] | ||||||||
Desulfuromonas michiganensis sp. Nov | 6.8 to 8.0 | 7.0 to 7.5 | Sung et al., 2003[29] | ||||||||
Geobacter lovleyi SZ | 6.5 to 7.2 | Sung et al., 2006[30] | |||||||||
(adapted from Damborský, 1999[31]; Yang et al. 2017a[2]) |
Impact on Dechlorination Rates
Lacroix et al. (2014)[32] examined the effect of pH on dechlorination rates for several organohalide-respiring consortia including SL2-PCEa, SL2-PCEb, AQ-1, and PM. Dechlorination rates as a function of pH (RpH) were fit to the relationship. File:Borden1w2 Equation 1.PNG
where Rmax is the maximum dechlorination rate at the optimal pH (pHopt), and n and σ are empirical fitting parameters (Table 2). Graphs of relative dechlorination rate (RpH / Rmax) are shown in Figure 1. Dechlorination of PCE to cDCE can continue at pH below 5.5. However, dechlorination of cDCE to VC and VC to ethene is severely inhibited below a pH of 5.5.
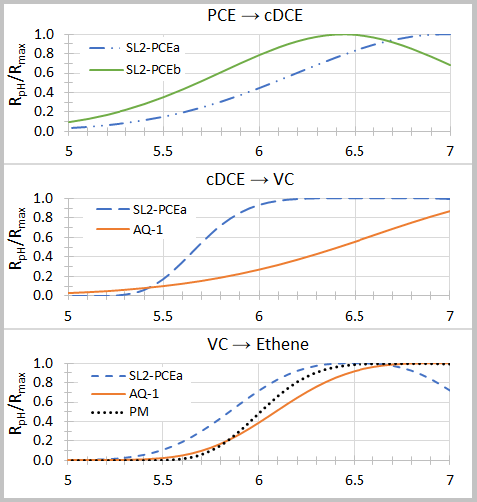
Low pH Tolerant Enrichments
Multiple researchers have attempted to develop enrichment cultures capable of complete dechlorination of PCE and TCE to ethene at low pH. However, to date, these attempts have had only modest success.
Rowlands (2004) [9] reported that the KB-1™ bioaugmentation culture has an optimal range of 6.0-8.3 and is completely inhibited below pH~5.0. Li (2012)[1] found that rates of VC reduction to ethene by a KB-1 culture declined by a factor of two as the pH was reduced from 7 to 6. Ethene production rates declined slowly over time and multiple degradation cycles. The decline in ethene production rates was accompanied by a decline of Dehalococcoides (Dhc) numbers, increase in methanogens, and a shift in total electron equivalents consumed in dechlorination towards methanogenesis. In methanol fed pH 6 cultures, ethene production rates slowly increased over hundreds of days, with one culture showing sustained VC dechlorination at pH 5.7 (Li, 2012)[1].
Using a bioaugmentation culture enriched from Savannah River Site aquifer material Eaddy (2008)[11] found that dechlorination of PCE and TCE slowed at a pH of 6.0 with increased accumulation of cDCE and VC. At pH 5.5, reduction of cDCE to VC and VC to ethene was completely inhibited.
Yang (2017a)[2] found that various dechlorinating pure cultures and the BDI consortium showed the highest dechlorination rates at circumneutral pH. Sulfurospirillum multivorans was the only organisms identified that dechlorinated PCE to cDCE at pH 5.5. Yang (2016)[33] and Yang et al. (2017a)[2] found that only one enrichment culture containing Desulfovibrio, Sulfurospirillum, and Megasphaera showed dechlorination of PCE to cDCE after repeated transfers at pH 5.5. After 40-days exposure to pH=5.5, Dhc lost the ability to dechlorinate VC to ethene (Yang et al. 2017b)[3].
Overall, these results indicate that: (a) dechlorination rates are highest at circumneutral pH; (b) certain microorganisms can grow while dechlorinating PCE and TCE at pH values of 5.5 and lower; and (c) some Dhc-containing consortia can dechlorinate cDCE and VC to ethene during short-term exposure to pH 5.5. However, at pH 5.5, the consortia do not grow and gradually lose their ability to dechlorinate VC to ethene. As a result, complete dechlorination to ethene will be inhibited at low pH. In addition, several groups are continuing work on development of improved enrichment cultures that are effective at low pH.
Summary
Acids are produced during ERD that can cause pH to decline, inhibiting rapid dechlorination. Reduction of PCE to TCE to cDCE can occur at pH down to 5.5 or lower. However, rates of cDCE reduction to VC to ethene are reduced below a pH of 6.0. For efficient dechlorination to non-toxic end products, the aquifer pH should be maintained above 6.0 during ERD.
References
- ^ 1.0 1.1 1.2 Li, Y.X., 2012. Adaptation of a Dechlorinating Culture, KB-1, to Acidic Environments. M.S. Thesis, University of Toronto, Toronto, ON, Canada. Report.pdf
- ^ 2.0 2.1 2.2 2.3 2.4 2.5 2.6 Yang, Y., Cápiro, N.L., Marcet, T.F., Yan, J., Pennell, K.D. and Loffler, F.E., 2017. Organohalide respiration with chlorinated ethenes under low pH conditions. Environmental Science & Technology, 51(15), pp.8579-8588. doi: 10.1021/acs.est.7b01510
- ^ 3.0 3.1 Yang, Y., Cápiro, N.L., Yan, J., Marcet, T.F., Pennell, K.D. and Löffler, F.E., 2017. Resilience and recovery of Dehalococcoides mccartyi following low pH exposure. FEMS microbiology ecology, 93(12), p.fix130. doi: 10.1093/femsec/fix130. Report.pdf
- ^ AFCEE, NFESC, ESTCP, 2004. Principles and Practices of Enhanced Anaerobic Bioremediation of Chlorinated Solvents. Parsons Corporation. Air Force Center for Environmental Excellence, Naval Facilities Engineering Service Center, and Environmental Security Technology Certification Program. Report PDF
- ^ ITRC, 2008. Enhanced attenuation of chlorinated organics (EACO-1): A decision framework for site transition. Washington, D.C.: Interstate Technology & Regulatory Council, Enhanced Attenuation: Chlorinated Organics Team Report pdf
- ^ Stroo, H.F., West, M.R., Kueper, B.H., Borden, R.C., Major, D.W. and Ward, C.H., 2014. In Situ Bioremediation Of Chlorinated Ethene Source Zones. In Chlorinated Solvent Source Zone Remediation (pp. 395-457). Springer New York. doi:10.1007/978-1-4614-6922-3_12
- ^ Vogel, T.M. and McCarty, P.L., 1985. Biotransformation of tetrachloroethylene to trichloroethylene, dichloroethylene, vinyl chloride, and carbon dioxide under methanogenic conditions. Applied and Environmental Microbiology, 49(5), pp.1080-1083.
- ^ Mohn, W.W. and Tiedje, J.M., 1992. Microbial reductive dehalogenation. Microbiological reviews, 56(3), pp.482-507.
- ^ 9.0 9.1 Rowlands, D., 2004. Development of optimal pH for degradation of chlorinated solvents by the KB-1TM anaerobic bacterial culture. M.S. Thesis, University of Guelph, Guelph, ON, Canada
- ^ 10.0 10.1 Vainberg, S., Steffan, R.J., Rogers, R., Ladaa, T., Pohlmann, D. and Leigh, D., 2006. Production and application of large-scale cultures for bioaugmentation. Proc 5th Internat Conf Remediation of Chlorinated and Recalcitrant Compounds, Monterey, CA, USA. May 22-25. Paper No. A-50.
- ^ 11.0 11.1 Eaddy, A., 2008. Scale-up and characterization of an enrichment culture for bioaugmentation of the P-area chlorinated ethene plume at the Savannah River site. M.S. Thesis, Clemson University, Clemson, SC, USA. Report.pdf
- ^ Duhamel, M., Wehr, S.D., Yu, L., Rizvi, H., Seepersad, D., Dworatzek, S., Cox, E.E. and Edwards, E.A., 2002. Comparison of anaerobic dechlorinating enrichment cultures maintained on tetrachloroethene, trichloroethene, cis-dichloroethene and vinyl chloride. Water Research, 36(17), pp.4193-4202. doi: 10.1016/S0043-1354(02)00151-3
- ^ McCarty, P.L., Chu, M.Y. and Kitanidis, P.K., 2007. Electron donor and pH relationships for biologically enhanced dissolution of chlorinated solvent DNAPL in groundwater. European Journal of Soil Biology, 43(5-6), pp.276-282. doi: 10.1016/j.ejsobi.2007.03.004. Report.pdf
- ^ 14.0 14.1 Lowe, S.E., Jain, M.K. and Zeikus, J.G., 1993. Biology, ecology, and biotechnological applications of anaerobic bacteria adapted to environmental stresses in temperature, pH, salinity, or substrates. Microbiological reviews, 57(2), pp.451-509
- ^ Slonczewski, J.L., 2009 Stress Responses: pH, In Encyclopedia of Microbiology (3rd Ed.), Editor-in-Chief: Schaechter, M., Academic Press: Oxford Vol 5. pp. 477-484
- ^ Foster, J.W., 1999. When protons attack: microbial strategies of acid adaptation. Current opinion in microbiology, 2(2), pp.170-174. doi: 10.1016/S1369-5274(99)80030-7
- ^ 17.0 17.1 Löffler, F.E., Yan, J., Ritalahti, K.M., Adrian, L., Edwards, E.A., Konstantinidis, K.T., Müller, J.A., Fullerton, H., Zinder, S.H. and Spormann, A.M., 2013. Dehalococcoides mccartyi gen. nov., sp. nov., obligately organohalide-respiring anaerobic bacteria relevant to halogen cycling and bioremediation, belong to a novel bacterial class, Dehalococcoidia classis nov., order Dehalococcoidales ord. nov. and family Dehalococcoidaceae fam. nov., within the phylum Chloroflexi. International journal of systematic and evolutionary Microbiology, 63(2), pp.625-635. doi: 10.1099/ijs.0.034926-0 [media: 2013-Loffler-Dehalococcoides_mccartyi_gen._nov....pdf| Report.pdf]
- ^ Zhuang, P. and Pavlostathis, S.G., 1995. Effect of temperature, pH and electron donor on the microbial reductive dechlorination of chloroalkenes. Chemosphere, 31(6), pp.3537-3548. doi:10.1016/0045-6535(95)00204-L
- ^ Vainberg, S., Condee, C.W. and Steffan, R.J., 2009. Large-scale production of bacterial consortia for remediation of chlorinated solvent-contaminated groundwater. Journal of Industrial Microbiology & Biotechnology, 36(9), pp.1189-1197. doi: 10.1007/s10295-009-0600-5
- ^ Holliger, C., Hahn, D., Harmsen, H., Ludwig, W., Schumacher, W., Tindall, B., Vazquez, F., Weiss, N. and Zehnder, A.J., 1998. Dehalobacter restrictus gen. nov. and sp. nov., a strictly anaerobic bacterium that reductively dechlorinates tetra-and trichloroethene in an anaerobic respiration. Archives of Microbiology, 169(4), pp.313-321. doi: 10.1007/s002030050577
- ^ Neumann, A., Scholz-Muramatsu, H. and Diekert, G., 1994. Tetrachloroethene metabolism of Dehalospirillum multivorans. Archives of Microbiology, 162(4), pp.295-301. ISSN: 0302-8933, 1432-072X doi: 10.1007/BF00301854
- ^ Scholz-Muramatsu, H., Neumann, A., Meßmer, M., Moore, E. and Diekert, G., 1995. Isolation and characterization of Dehalospirillum multivorans gen. nov., sp. nov., a tetrachloroethene-utilizing, strictly anaerobic bacterium. Archives of Microbiology, 163(1), pp.48-56. doi: 10.1007/BF00262203
- ^ Utkin, I., Woese, C. and Wiegel, J., 1994. Isolation and characterization of Desulfitobacterium dehalogenans gen. nov., sp. nov., an anaerobic bacterium which reductively dechlorinates chlorophenolic compounds. International Journal of Systematic and Evolutionary Microbiology, 44(4), pp.612-619. doi: 10.1099/00207713-44-4-612
- ^ Gerritse, J., Renard, V., Gomes, T.P., Lawson, P.A., Collins, M.D. and Gottschal, J.C., 1996. Desulfitobacterium sp. strain PCE1, an anaerobic bacterium that can grow by reductive dechlorination of tetrachloroethene or ortho-chlorinated phenols. Archives of microbiology, 165(2), pp.132-140. doi: 10.1007/s002030050308
- ^ Fogel, S., Findlay, M., Folsom, S. and Kozar, M., 2009. The importance of pH in reductive dechlorination of chlorinated solvents. In Proceedings Tenth International In Situ and On-Site Bioremediation Symposium, Baltimore, MD, USA, May(pp. 5-8)
- ^ Krumholz, L.R., Sharp, R. and Fishbain, S.S., 1996. A freshwater anaerobe coupling acetate oxidation to tetrachloroethylene dehalogenation. Applied and Environmental Microbiology, 62(11), pp.4108-4113. doi:10.1128/AEM.01380-18
- ^ Krumholz, L.R., 1997. Desulfuromonas chloroethenica sp. nov. uses tetrachloroethylene and trichloroethylene as electron acceptors. International Journal of Systematic and Evolutionary Microbiology, 47(4), pp.1262-1263. doi: 10.1099/00207713-47-4-1262
- ^ DeWeerd, K.A., Mandelco, L., Tanner, R.S., Woese, C.R. and Suflita, J.M., 1990. Desulfomonile tiedjei gen. nov. and sp. nov., a novel anaerobic, dehalogenating, sulfate-reducing bacterium. Archives of Microbiology, 154(1), pp.23-30
- ^ Sung, Y., Ritalahti, K.M., Sanford, R.A., Urbance, J.W., Flynn, S.J., Tiedje, J.M. and Löffler, F.E., 2003. Characterization of two tetrachloroethene-reducing, acetate-oxidizing anaerobic bacteria and their description as Desulfuromonas michiganensis sp. nov. Applied and Environmental Microbiology, 69(5), pp.2964-2974. doi 10.1128/AEM.69.5.2964–2974.2003. Report.pdf
- ^ Sung, Y., Fletcher, K.E., Ritalahti, K.M., Apkarian, R.P., Ramos-Hernández, N., Sanford, R.A., Mesbah, N.M. and Löffler, F.E., 2006. Geobacter lovleyi sp. nov. strain SZ, a novel metal-reducing and tetrachloroethene-dechlorinating bacterium. Applied and Environmental Microbiology, 72(4), pp.2775-2782. doi: 10.1128/AEM.72.4.2775-2782.2006
- ^ Damborský, J., 1999. Tetrachloroethene-dehalogenating bacteria. Folia microbiologica, 44(3), pp.247-262. doi: 10.1007/BF02818543
- ^ 32.0 32.1 32.2 Lacroix, E., Brovelli, A., Barry, D.A. and Holliger, C., 2014. Use of silicate minerals for pH control during reductive dechlorination of chloroethenes in batch cultures of different microbial consortia. Applied and environmental microbiology, pp.AEM-00493. doi: 10.1128/AEM.00493-14
- ^ Yang, Y., 2016. Bioremediation of Chlorinated Ethenes: pH Effects, Novel Dechlorinators and Decision-Making Tools. Ph.D. Dissertation, University of Tennessee, Knoxville, TN, USA. Report.pdf